2.3. The Detector's time behaviour
A detector’s time resolution is limited by its response to an instantaneous change of the input signal. Due to electrical capacities of the light sensitive element and the electronics, the output signal does not change instantaneously but gradually increases or decreases until it reaches its final value. The detector’s rise time is defined as the time span required for the output signal to rise from a certain low percentage (usually 10 %) to a certain high percentage (usually 90 %) level of the maximum value when a steady input is instantaneously applied. The fall time is similarly defined as the time span required for the output signal to drop from a certain high percentage (usually 90 %) to a certain low percentage (usually 10 %) of the maximum value when a steady input is instantaneously removed.
Typically, the detector’s response to an instantaneous change of the input signal approaches the final value exponentially. The detector’s time behavior is thus best described by the time constant τ, which is the time span required for the output signal to vary from its initial value by 63 % of its final change (the value of 63 % is derived from 1 – 1/e, which equals 0.63). The temporal change of the output signal Y(t) from its initial value Y0 to its final value Yf is therefore given by
Y(t) = Y0 + ( Yf - Y0 ) × e t / τ
Gigahertz Optik’s integral detectors use photodiodes that are typically characterized by time constants in μs. Since most variable light sources change their intensity levels in significantly longer time scales, the detector’s time constant is not really an issue for most applications. However, lasers in particular are often pulsed with a frequency in the order of 109 Hz (for example in telecommunication), which corresponds to signal periods in the order of 1 ns. Here, the relatively slow response of normal photodiodes prevents the accurate characterization of the laser signal’s time characteristics.
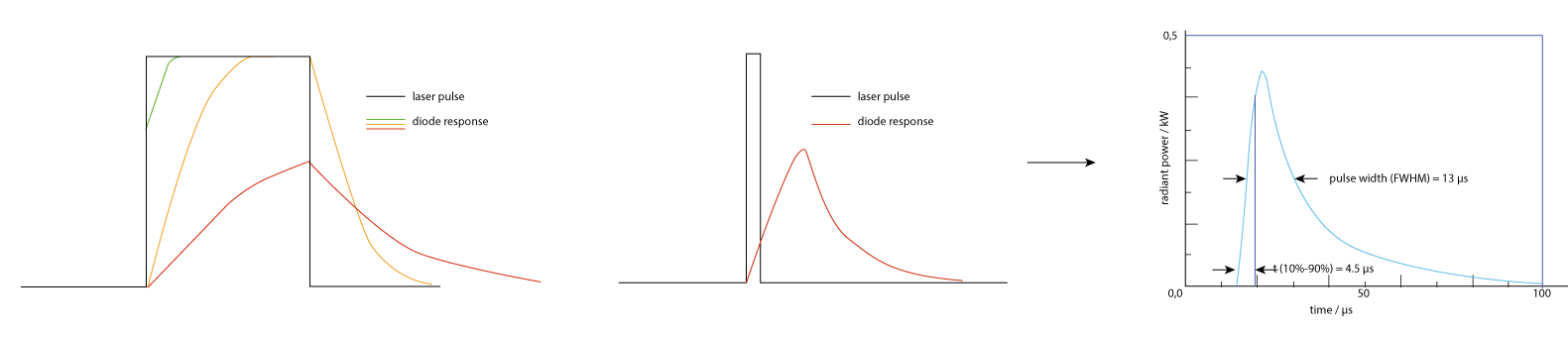
The challenge of measuring pulsed radiation is to attenuate the integrating sphere detectors according to the peak pulse power or to choose the sphere size accordingly. The rise time of the photodiode and any pulse broadening that may occur due to the multiple reflections caused by the integrating sphere must be taken into account.
Two photodiode systems are also used in order to meet the high time requirements for fast, time-resolved pulse measurement as well as precise pulse power measurement.
For the precise measurement of the pulse power of short pulses the so called pulse stretching method can be used. For this approach the optometer (current amplifier) has a rather long (e.g. 20 ms) standard time constant in all gain ranges. Thus short light pulses are stretched to 20 ms. Resulting the pulse energy of the stretched pulse can then be measured precisely with a e.g.100 µs sample rate of the optometer. This is needed since most optometer can't sample in sub-ns range.
In some cases, the sphere entry port needs to be e.g. 7 mm in accordance with eye safety. Sometimes specific geometries or accessories are needed in order to fulfill the application. In the following you will find information on the application of laser measurement as well as laser pulse measurement and corresponding products.